Mechanisms of PLD
The principle of pulsed laser deposition, in contrast to the simplicity of the system set-up, is a very complex
physical phenomenon. It involves all the physical processes of laser-material interaction during the impact of
the high-power pulsed radiation on a solid target. It also includes the formation of the plasma plume with high
energetic species, the subsequent transfer of the ablated material through the plasma plume onto the heated
substrate surface and the final film growth process. Thus PLD generally can be divided into the following four
stages.
- Laser radiation interaction with the target
- Dynamic of the ablation materials
- Decomposition of the ablation materials onto the substrate
- Nucleation and growth of a thin film on the substrate surface
In the first stage, the laser beam is focused onto the surface of the target. At sufficiently high energy density
and short pulse duration, all elements in the target surface are rapidly heated up to their evaporation temperature.
Materials are dissociated from the target and ablated out with stoichiometry as in the target. The instantaneous
ablation rate is highly dependent on the fluences of the laser irradiating on the target. The ablation mechanisms
involve many complex physical phenomena such as collisional, thermal and electronic excitation, exfoliation and
hydrodynamics.
During the second stage the emitted materials tend to move towards the substrate according to the laws of gas-dynamic
and show the forward peaking phenomenon [1]. R.K. Singh [2] reported that the spatial thickness varied as a
function of cosnphi , where n>>1. The laser spot size and the plasma temperature have significant
effects on the deposited film uniformity. The target-to-substrate distance is another parameter that governs the
angular spread of the ablated materials. Hanabusa [3] also found that a mask placed close to the substrate could
reduce the spreading.
The third stage is important to determine the quality of thin film. The ejected high-energy species impinge onto the
substrate surface and may induce various type of damage to the substrate. The mechanism of the interaction is
illustrated in the following figure. These energetic species sputter some of the surface atoms and a collision region
is established between the incident flow and the sputtered atoms. Film grows immediately after this thermalized
region (collision region) is formed. The region serves as a source for condensation of particles. When the condensation
rate is higher than the rate of particles supplied by the sputtering, thermal equilibrium condition can be reached
quickly and film grows on the substrate surface at the expense of the direct flow of the ablation particles.
Nucleation-and-growth of crystalline films depends on many factors such as the density, energy, degree of ionization,
and the type of the condensing material, as well as the temperature and the physical-chemical properties of the
substrate. The two main thermodynamic parameters for the growth mechanism are the substrate temperature T and the
supersaturation m. They can be related by the following equation:
Dm = kT ln(R/Re)
where k is the Boltzmann constant, R is the actual deposition rate, and Re is the equilibrium
value at temperature T.
The nucleation process depends on the interfacial energies between the three phases present - substrate, the condensing
material and the vapour. The minimum-energy shape of a nucleus is like a cap. The critical size of the nucleus depends
on the driving force, i.e. the deposition rate and the substrate temperature. For the large nuclei, a characteristic
of small supersaturation, they create isolate patches (islands) of the film on the substrates, which subsequently grow
and coalesce together. As the supersaturation increases, the critical nucleus shrinks until its height reaches an atomic
diameter and its shape is that of a two-dimensional layer. For large supersaturation, the layer-by-layer nucleation
will happen for incompletely wetted foreign substrates.
The crystalline film growth depends on the surface mobility of the adatom (vapour atoms). Normally, the adatom will
diffuse through several atomic distances before sticking to a stable position within the newly formed film.
The surface temperature of the substrate determines the adatom's surface diffusion ability. High temperature favours
rapid and defect free crystal growth, whereas low temperature or large supersaturation crystal growth may be
overwhelmed by energetic particle impingement, resulting in disordered or even amorphous structures.
Metev and Veilo [4, 5] suggested that the N99, the mean thickness at which the growing,
thin and discontinuous film reaching continuity is given by the formula:
N99 = A(1/R)1/3 exp (-1/T),
where R is the deposition rate (supersaturation related) and T is the temperature of the substrate
and A is a constant related to the materials.
In the PLD process, due to the short laser pulsed duration (~10 ns) and the small temporal spread (<=10exp(-6) s) of
the ablated materials, the deposition rate can be enormous (~10exp(-6)m/s). Consequently a layer-by-layer nucleation is
favoured and ultra-thin and smooth film can be produced. In addition the rapid deposition of the energetic ablation
species helps to raise the substrate surface temperature. In this respect PLD tends to demand a lower substrate
temperature for crystalline film growth.
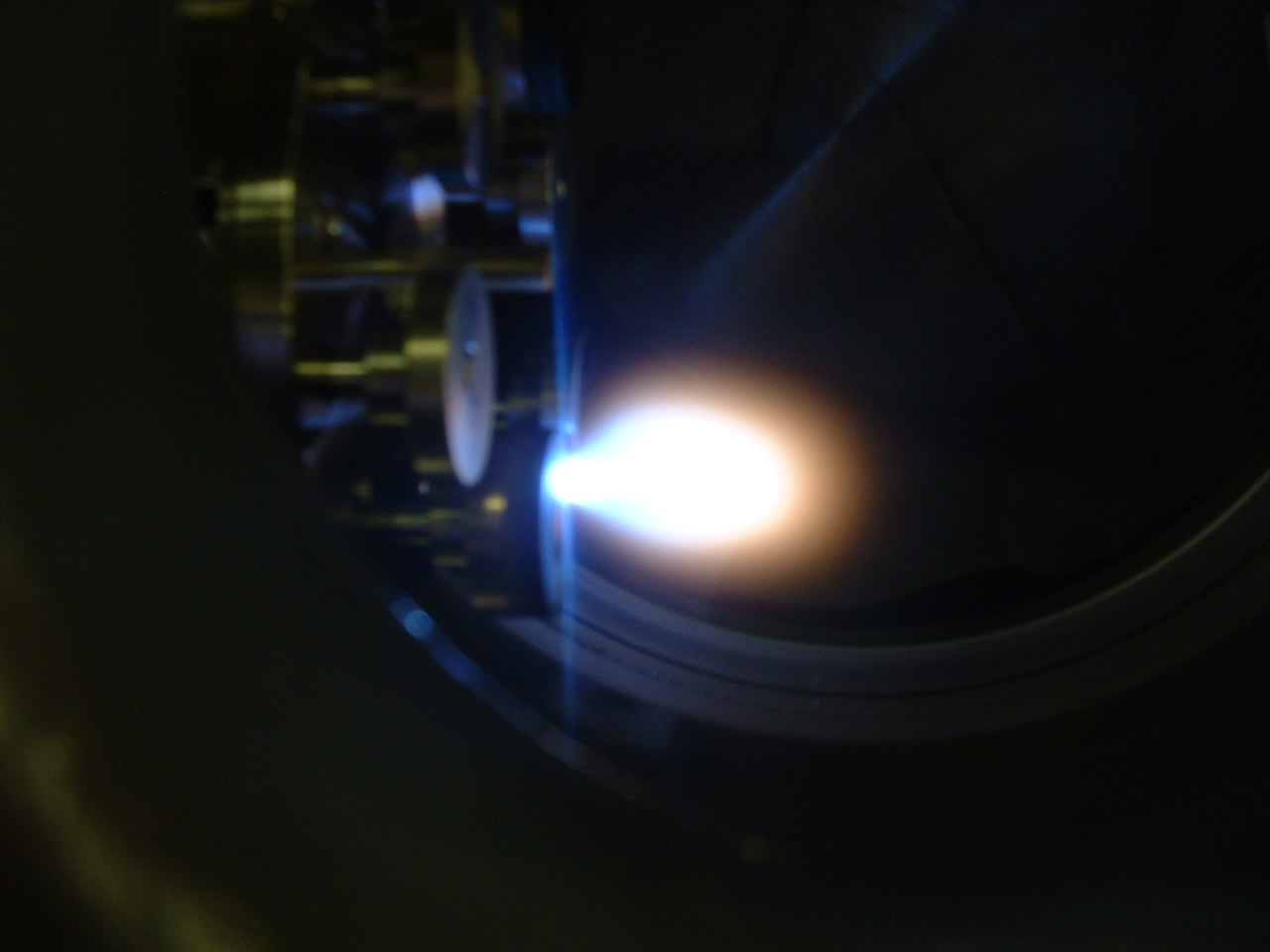
Another plasma plume
References:
- A. Namiki, T. Kawai, K. Ichige, Surf. Sci. 166, 129 (1986)
- R.K. Sing, SPIE 2945, 10
- M. Hanabusa, Mater. Res. Soc. Symp. Proc. 285, 447 (1993)
- T.J. Goodwin, V.L. Leppert, S.H. Risbud, I.M. Kennedy, and H.W.H. Lee, Appl. Phys. Lett. 10, 3122 (1997)
- S. Metev, K. Meteva, Appl. Surf. Sci. 43, 402 (1989)
>