The crystal structure of NKN
Sodium potassium niobate (NKN) is the second of the two most commonly investigated lead-free ferroelectric
systems today, the other first one being bismuth sodium titanate-barium titanate (BNT-BT).
KNN is the only known lead-free system with a perovskite structure, which has a higher Curie temperature
than the commonly used lead zirconate titanate (PZT) and piezoelectric properties of the same order of magnitude.
High chemical inertness and compatibility with human tissue allow for medical uses as well.
Its high electromechanical coupling factor and low dielectric constant make it interesting for ultrasonic
applications[1]. Following the work of Saito et al.[2,3], the research effort into NKN-based materials in fact
surpassed that of BNT-based materials in 2005. A few drawbacks, however, still prevent wide scale industrial use
today. NKN is made from volatile alkali metal compounds, which require carefully controlled manufacturing
conditions and low reaction temperatures. Furthermore, NKN exhibits poor densification behaviour in pressureless
sintering conditions. There is also a phase transition between two ferroelectric phases above room temperature.
Heating above this transition temperature causes accelerated aging.
Sodium potassum niobate (NKN) is a ferroelectric system with a complex perovskite structure. Complex in this
case refers to the fact that the A-site is shared by more than one type of ion. NKN is a mixed system of orthorhombic
ferroelectric potassium niobate (KN) displaying similar phase transitions (see Fig 14) to barium titanate but at higher
temperatures[4,5] and orthorhombic sodium niobate (NN), which was originally reported as ferroelectric[6] but is in
fact antiferroelectric[7]. NKN was first reported as ferroelectric by Shirane et al.[4], though without identifying
the enhanced properties at the 50/50 potassium to sodium ratio. Egerton et al.[8] reported the first piezoelectric
data and identified enhanced ferroelectric properties at the 50 % potassium niobate composition.
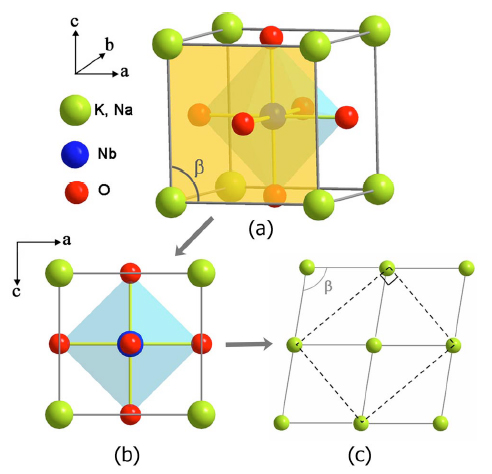
The crystal structure of NKN
The structure illustration for NKN at room temperature is shown in the figure above. Although the structure of NKN
is orthorhombic at room temperature, the perovskite type ABO3 subcell, as shown in (a), possesses a
monoclinic symmetry, with lattice parameters am=cm>bm while bm
is perpendicular to the amcm plane and the angle beta is a little bit more than 90°. For
the composition (Na0.5K0.5)NbO3 no superlattice reflections were found in previous
XRD measurements so no cornerlinked O octahedra tilting should be taken into account.
The projection view of the subcell along the bm axis is shown in (b). Since the angle beta is very close to
90°, it looks like that the am axis is also perpendicular to the cm axis. Therefore, in order to
show up the geometrical relationship more clearly, we could exaggerate the angle beta much more than 90° and draw
the projection of four adjacent perovskite subcells together, but omit the Nb and O atoms, as shown in (c).
As the am axis equals the cm axis in length, the diagonals linked by dashed lines in (c)
form a rectangle, which is the projection of the unit cell of NKN along the bm axis. Hence it is be easy to
understand that the perovskite type subcell of NKN is monoclinic while its unit cell has orthorhombic symmetry at
room temperature.
The phase diagram of NKN
NaxK1-xNbO3 has several thermally induced phase transitions
and morphotropic phase boundaries (MPBs) summarised by Ahtee et al.[9,10] as displayed in the figure above. (For comparison
see here the phase diagram of PZT.)
Regions labelled with Q, K, and L are monoclinic ferroelectric, M, G is orthorhombic
ferroelectric; F, H and J are tetragonal ferroelectric. Region P is orthorhombic antiferroelectric.
At room temperature, MPBs lie at 17.5 %, 32.5 % and 47.5 % NN content. Notable is the almost composition independent
phase transition temperature between ferroelectric phases at ~200 °C and between ferroelectric and paraelectric
phases at ~400 °C (in contrast to the composition dependent transition temperature of PZT). Only small substitutions of sodium for potassium in NaNbO3 cause a transition to ferroelectric
(Region Q) from the pure antiferroelectric sodium niobate (Region P).
References:
- H. Birol, D. Damjanovic, and N. Setter, "Preparation and characterization of (K0.5Na0.5)NbO3 ceramics,"
Journal of the European Ceramic Society, 26[6] 861-6 (2006).
- Y. Saito, H. Takao, T. Tani, T. Nonoyama, K. Takatori, T. Homma, T. Nagaya, and M. Nakamura,
"Lead-free piezoceramics," Nature, 432[7013] 84-7 (2004).)
- I. Fuyuno, "Toyota's production line leads from lab to road," Nature, 435[7045] 1026-7 (2005).
- G. Shirane, R. Newnham, and R. Pepinsky, "Dielectric properties and phase transitions of NaNbO3 and
(Na,K)NbO3," Physical Review, 96[3] 581-8 (1954).
- G. Shirane, H. Danner, A. Pavlovic, and R. Pepinsky, "Phase Transitions in Ferroelectric KNbO3,
"Physical Review, 93[4] 672-3 (1954)
- B. T. Matthias and J. P. Remeika, "Dielectric properties of sodium and potassium niobates,
"Physical Review, 82[5] 727-9 (1951).
- E. A. Wood, "Polymorphism in potassium niobate, sodium niobate, and other ABO3 Compounds,
"Acta Crystallographica, 4[4] 353-62 (1951)
- L. Egerton and D. M. Dillon, "Piezoelectric and dielectric properties of ceramics in the system potassium sodium niobate,"
Journal of the American Ceramic Society, 42[9] 438-42 (1959)
- M. Ahtee and A. M. Glazer, "Lattice parameters and tilted octahedra in sodium-potassium niobate solid solutions,"
Acta Crystallographica, Section A: Foundations of Crystallography, 32 434-45 (1976)
- M. Ahtee and A. W. Hewat, "Structural phase-transitions in sodium-potassium niobate solid-solutions by neutron powder
diffraction," Acta Crystallographica, Section A: Foundations of Crystallography, 34[Mar] 309-17 (1978)
>